Benzene Structure and Properties Chemistry Tutorial
Key Concepts
Please do not block ads on this website.
No ads = no money for us = no free stuff for you!
Discovery of Benzene
In 1825, Michael Faraday isolated a compound, benzene, from the oil which collected in the gas pipes in London and determined that it had an empirical formula of CH.
In 1834, Eilhardt Mitscherlich synthesized benzene and determined its molecular formula to be C6H6.
In 1858, Kekulé proposed that carbon atoms could join to one another to form chains.
So, a number of different structures with the molecular formula C6H6 could be drawn, some of which are shown below:
possible straight-chain structures: |
|
CH2=CH-C≡C-CH=CH2 |
|
CH3-C≡C-C≡C-CH3 |
possible ring structures: |
|
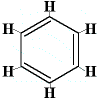 |
|
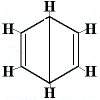 |
It would take many decades before Chemists began to understand the molecular structure of benzene.
Kekulé Structure for Benzene
Benzene was known to have the molecular formula C6H6, but its structural formula was unknown.
If benzene was a straight-chain unsaturated hydrocarbon it should readily undergo addition reactions (just like other alkenes and alkynes).
For example, bromine, Br2, should add across the double or triple bond as shown below:
possible straight-chain structure addition reaction: |
CH3-C≡C-C≡C-CH3 |
+ Br2 |
→ |
CH3-CBr=CBr-C≡C-CH3 |
But, when bromine is added to benzene at 25°C and 1 atm (101.3 kPa) pressure, no reaction seems to occur.
So, the possible structures for benzene involving double and/or triple bonds arranged in a chain seemed most unlikely as these should undergo addition reactions.
If bromine is added to benzene in the presence of FeBr3, it undergoes a substitution reaction in which one of the hydrogen atoms is replaced by a bromine atom to produce a compound with the formula C6H5Br, as shown below:
known substitution reaction: |
C6H6 |
|
+ Br2 |
|
FeBr3 → |
|
C6H5Br |
|
+ HBr |
In this respect, benzene was behaving much more like a saturated hydrocarbon (only single bonds between carbon atoms).
So, the first structure proposed by Kekulé for benzene in 1865 did not contain any double bonds but did arrange the 6 carbon atoms in a ring as shown below:
Kekulé realised that this molecule breaks the tetravalence rule for carbon, that is, each carbon atom is forming only 3 bonds rather than 4.
So, in 1872 Kekulé proposed a benzene structure composed of three alternate single and double bonds as shown below:
Please note that although the carbon atoms are not written within the structure, it is always understood that a cabon atom exists where two lines of the hexagon join, so that there are 6 "joins", 6 angles, and therefore 6 carbon atoms.
In this structure each carbon atom is bonded to a hydrogen atom, and, to two other carbon atoms. One of the carbon-carbon bonds is a single bond, the other carbon-carbon bond is a double bond. Each carbon atom has made 4 bonds.
While this structure does not explain why benzene does not undergo addition reactions, it could explain why benzene forms only one structural isomer when benzene reacts with bromine in a substitution reaction as shown below:
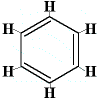 proposed benzene structure |
|
+ Br2 |
|
→ |
|
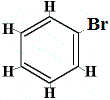 only 1 structural isomer |
|
+ HBr |
The other proposed ring structures for benzene would have predicted more than one structural isomer for the product of the substitution reaction as shown below:
other proposed ring structure reaction: |
|
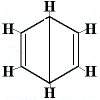 |
|
Br2 → |
|
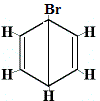 structural isomer 1 |
|
+ |
|
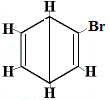 structural isomer 2 |
What would happen if another hydrogen atom were replaced (substituted) with a bromine atom?
C6H5Br |
|
+ Br2 |
|
FeBr3 → |
|
C6H4Br2 |
|
+ HBr |
How many structural isomers of C6H4Br2 would be produced?
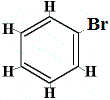 |
Br2 → FeBr3 |
|
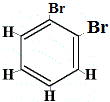 structural isomer 1 |
+ |
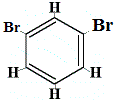 structural isomer 2 |
+ |
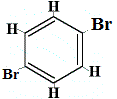 structural isomer 3 |
+ |
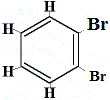 structural isomer 4 |
Structural isomers 1 and 4 are different because in isomer 1 the two Br atoms are bonded to carbon atoms on a C-C bond, while in isomer 4 the two Br atoms are bonded to carbon atoms on C=C bond.
However, when the C6H5Br made from benzene reacts with bromine in a substitution reaction, only 3 structural isomers of C6H4Br2 are produced!
In order to resolve this problem, Kekulé proposed that the benzene ring is in rapid equilibrium between structures in which the double bonds are in the alternative positions as shown below:
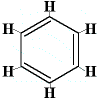 |
⇋ |
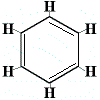 |
So, when bromine reacts with C6H5Br in a substitution reaction to produce C6H4Br2, pairs of structural isomers of C6H4Br2 are in equilibrium with each other.
This means that structural isomers 1 and 4 shown above, which only differ in that Br atoms are bonded across a single bond or a double bond, are now in rapid equilibrium with each other as shown below:
Br atoms on adjacent C atoms, structural isomer 1 has Br atoms across C-C structural isomer 4 has Br atoms across C=C |
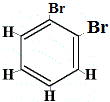 structural isomer 1 |
⇋ |
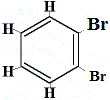 structural isomer 4 |
According to Kekulé, these two structural isomers of C6H4Br2 are in such rapid equilibrium that they cannot be separated out as two separate molecules, so it appears that there are only 3 isomers instead of 4.
While this rapid-equilibrium structure for benzene, known as the Kekulé structure, proved to be useful, it does not explain the unique stability (lack of reactivity) of the benzene ring, it does not explain why benzene does not readily undergo addition reactions but can be induced to undergo addition reactions.
Resonance Hybrid Structure for Benzene
The Kekulé structure for benzene meant that there were two distinct structures of C6H6 in rapid equilibrium with each other.
Each of these structures was made up of 3 carbon-carbon single bonds (C-C) and 3 carbon-carbon double bonds (C=C).
Carbon-carbon single bonds (C-C) have been found to be about 1.5 Å (1.5 × 10-10 m) in length.
Carbon-carbon double bonds (C=C) have been found to be about 1.3 Å (1.3 × 10-10 m) in length.
If the Kekulé structure for benzene was an accurate representation of a benzene molecule, then when the carbon-carbon bond lengths are measured we should find half the bonds are 1.5 Å and the other half are 1.3 Å.
But, we don't! We find that all the carbon-carbon bond lengths in benzene are all the same, they are all 1.4 Å, which is half-way between the length of a C-C bond and the length of a C=C bond.
In 1940 it was suggested that benzene is NOT made up of the two structures above in rapid equilibrium, but that each benzene molecule is resonating between the structures as shown below:
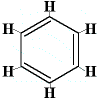 |
↔ |
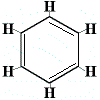 |
This means that the electrons making up the bonds in each single molecule of benzene are actually constantly in motion, producing the resonance hybrid structure which is the composite, or average, structure so that each carbon-carbon bond is identical and halfway between the length of a single and a double bound.
This resonance hybrid stucture for benzene can be depicted as a hexagon with dotted lines inside the solid lines to represent the idea that the electrons are in motion and that all the carbon-carbon bonds are identical(2) as shown below:
This resonance hybrid structure accounts for all the carbon-carbon bond lengths being the same.
It also accounts for there being only 3 structural isomers for C6H4Br2 molecules produced by substitution reactions with benzene, since all the carbon-carbon bonds in bond are represented as being truly identical.
It even goes a long way towards explaining why benzene does not readily undergo addition reactions, because it does not contain double bonds like the unsaturated hydrocarbons (alkenes and alkynes).
Molecular Orbital Theory Structure for Benzene
The electron configuration for a carbon atom is: 1s2 2s2 2p2
The first energy level is full (1s2) so the 1s electrons are not available for bonding.
The 4 electrons in the second energy (2s2 2p2) are the electrons that are available to take part in bonding.
There are a total of 4 atomic orbitals in the second energy level: one 2s orbital and three 2p orbitals (2px, 2py and 2pz). We can represent the electrons in a carbon atom that are available to make bonds in an orbital diagram as shown below:
↑ E N E R G Y
|
|
↑ |
|
↑ |
|
|
|
2p |
|
2p |
|
2p |
|
↑↓ |
|
|
|
|
|
2s |
|
|
|
|
|
Each arrow (↑ or ↓) represents an electron (spin up or spin down, spin quantum number +½ or -½).
Carbon does not use these 4 atomic orbitals in their pure states for bonding, instead, carbon hybridizes (blends) its second energy atomic orbitals to form hybrid molecular orbitals.
In benzene, carbon hybridizes its 2s orbital with two of its available 2p orbitals which produces three hybrid molecular orbitals which are called sp2 orbitals, leaving one 2p orbital unhybridized. The orbital diagram for the electron configuration of carbon atoms in the benzene molecule is shown below:
↑ E N E R G Y
|
|
|
|
|
|
↑ |
|
|
|
|
|
2p |
↑ |
|
↑ |
|
↑ |
|
sp2 |
|
sp2 |
|
sp2 |
|
|
The sp2 hybrid molecular orbitals are all of equal energy. This energy is greater than the original 2s atomic orbital but less than the energy of the 2p orbital. There is one electron in each of the three sp2 hybrid molecular orbitals, and one electron in the unhybridized 2p orbital.
The three sp2 hybrid molecular orbitals around each carbon nucleus in the benzene molecule will lie as far apart from one another as possible, that is, the three sp2 hybrid molecular orbitals lie in a plane with angles of 120° between them.
Each carbon atom in the benzene molecule uses its sp2 hybrid molecular orbitals to form covalent bonds, one of these electrons is used to bond to a hydrogen atom, one is used to bond to a carbon atom, and the last is used to bond to another carbon atom as shown below:
The resulting molecule is planar (or flat) with bond angles of 120°.
But what happens to the electron in the higher energy, unhybridized 2p orbital?
The 2p orbitals lie at right angles (are perpendicular) to the plane of the benzene molecule. One lobe of the 2p orbital lies above the plane of the benzene molecule and one lobe lies below the plane of the benzene molecule as shown below:
This enables the sides of the lobes of each 2p orbital to overlap the sides of the lobes of the adjacent 2p orbitals.
The electrons in the 2p orbitals are no longer held between just two carbon atoms, they are now spread out over the whole benzene ring. We say that these electrons are delocalised:
In structural formula, this "cloud" of mobile delocalised electrons is represented as a circle inscribed within the hexagon of carbon-carbon atoms making up benzene as shown below:
In this representation, a carbon atom exists at each angle of the hexagon so there are 6 carbon atoms and the hydrogen atoms are not shown but it is understood that each carbon atom is covalently bonded to 1 hydrogen atom.
This the most commonly used representation for benzene in structural formula, however, you will find that the Kekulé structure is often used when showing how a chemical reaction involving benzene occurs.
Footnotes:
(1) The term 'aromatic' was originally applied to benzene and related compounds because of their distinctive odours or aromas. Today the term is applied to compounds that contain cyclic clouds of delocalised π electrons above and below the plane of the molecule.
(2) This structure for benzene was actually first used by Johannes Theile in 1899 who used a broken circle to stand for partial bonds.